Doi, Kentaro
Affiliation | Department of Mechanical Engineering |
---|---|
Title | Professor |
Fields of Research | Micro- and Nano-Thermofluid Engineering, Quantum Molecular Dynamics |
Degree | Ph.D. |
Academic Societies | JSME, JPS, CSJ, JAP, and ACS |
doi@me Please append ".tut.ac.jp" to the end of the address above. |
|
Laboratory website URL | https://tfelab.jp |
Researcher information URL(researchmap) | Researcher information |
Research
We are interested in fabrication and measurement techniques related to thermofluidic phenomena of micro- and nanometer scales. In particular, we are conducting basic research with a view to engineering applications, regarding the functions of electron and ion transport phenomena in micro- and nanofluidic devices. Recent research topics are below:
(1) Measurements of local electric feilds, conductivity, and pH using glass microelectrodes
(2) Nonequilibrium ion transport phenomena in micro- and nanogaps
(3) Firts-principles molecular dynamics simulation for chmical reaction systems.
Theme1:Measurements of local electric feilds, conductivity, and pH using glass microelectrodes
Overview
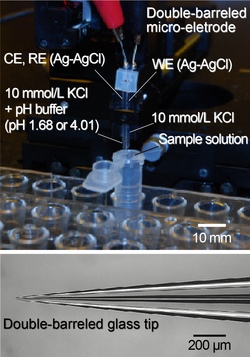
When an electric field is externally applied to a microchannel filled with an electrolyte solution, a weak ionic current is generated. The strength of the electric field changes depending on the structure of the channel, and charged tiny particles and biopolymers are transported by the electrostatic force of the electric field. In order to efficiently transport and detect particles, it is important to understand the electric field distributions inside fluidic channels. In this study, we measured local electric fields inside a channel by pulling the glass tube so that the tip diameter was 1 μm or less, and using a glass microelectrode in which an electrolyte solution and Ag-AgCl wire were inserted. Furthermore, we succeeded in identifying the salt concentration by measuring the conductivity. Also, the pH of sample solutions can be measured by making a double-barreled glass microelectrode (a bundle of two glass tubes) and filling one with a buffer solution and the other with an electrolyte solution.
Selected publications and works
[1] K. Doi, N. Asano, and S. Kawano, Development of Glass Micro-Electrodes for Local Electric Field, Electrical Conductivity, and pH Measurements, Sci. Rep. 10, 4110 (2020).
Keywords
Theme2:Nonequilibrium ion transport phenomena in micro- and nanogaps
Overview
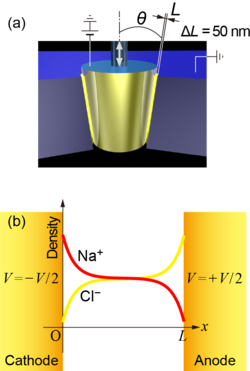
Ion transport phenomenon in a liquid is known to consist of electrophoretic (conduction), diffusion, and advection of ions. By investigating the unsteady phenomenon from immediately after a voltage is applied to the steady state, details of the ion transport phenomena in liquids can be elucidated, since each phenomenon has a different spatiotemporal scale. In the present study, the distance between electrodes was varied over a wide range from nm to mm, and the ionic current response to voltage application was theoretically predicted and measured experimentally. As a result, it was found that the electric field was strongly screened because the electrode surface was covered with ions immediately after the voltage was applied, and then the concentration field away from the electrode was slowly formed. This study clarified the dynamics of ion transport phenomena of micro- and nanoscales in detail.
Selected publications and works
[1] K. Doi, M. Ueda, and S. Kawano, Theoretical Model of Nanoparticle Detection Mechanism in Microchannel with Gating Probe Electrodes, J. Comput. Sci. Technol. 5 (2011), 78-88.
[2] K. Doi. M. Tsutsui, T. Ohshiro, C.-C. Chien, M. Zwolak, M. Taniguchi, T. Kawano, S. Kawano, and M. Di Ventra, Nonequilibrium Ionic Response of Biased Mechanically Controllable Break Junction (MCBJ) Electrodes, J. Phys. Chem. C 118 (2014), 3758-3765.
[3] K. Doi, A. Yano, and S. Kawano, Electrohydrodynamic Flow through a 1 mm2 Cross-Section Pore Placed in an Ion-Exchange Membrane, J. Phys. Chem. B 119 (2015), 228-237.
[4] S. Tanaka, M. Tsutsui, H. Theodore, H. Yuhui, A. Arima, T. Tsuji, K. Doi, S. Kawano, M. Taniguchi, and T. Kawai, Tailoring Particle Translocation via Dielectrophoresis in Pore Channels, Sci. Rep. 6, 31670 (2016).
Keywords
Theme3:Firts-principles molecular dynamics simulation for chmical reaction systems
Overview
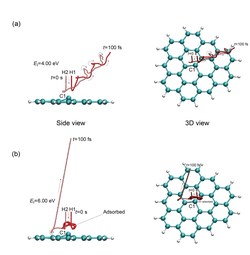
For example, the surface of a two-dimensional structure composed of carbon atoms such as graphene is known to be covered by a cloud of electrons. When atoms or molecules collide with such a stable surface, they will repel. On the other hand, if the surface of graphene is slightly distorted or if other elements are added, the cloud of electrons may be modulated, causing the transfer of electrons between the carbon atoms and the colliding atoms or molecules and resulting in adsorption. In conventional molecular dynamics simulations, it is difficult to simulate chemical reaction systems because the electron transfer is not taken into consideration. It enabled molecular dynamics simulation with movement. As a result, we succeeded in simulating a series of processes in which colliding hydrogen molecules are dissociated and further absorbed on the graphene surface, which has a partially steric structure, as shown in the figure. This makes it possible to simulate both chemisorption and physisorption phenomena of hydrogen molecules on the surfaces, and is expected to be applied to the design of hydrogen storage materials.
Selected publications and works
[1] H. Nakano, H. Ohta, A. Yokoe, K. Doi, and A. Tachibana, First-principle molecular-dynamics study of hydrogen adsorption on an aluminum-doped carbon nanotube, J. Power Sources 163 (2006), 125-134.
[2] A. Fukushima, A. Sawairi, K. Doi, M. Senami, L. Chen, H. Cheng, and A. Tachibana, Role of an aluminum atom on graphene for hydrogen adsorption, J. Phys. Soc. Jpn. 80 (2011) 074705.
[3] K. Doi, I. Onishi, and S. Kawano, Dissociative adsorption of H2 molecules on steric graphene surface: Ab initio MD study based on DFT, Comput. Theor. Chem. 994 (2012), 54-64.
Keywords
Title of class
Introduction of Mechanical Engineering (B11510090), Applied Mathematics 1 (B1161011, B1163019), Thermal Fluids Transport (B11630100), Transport Phenomena 1 (M21624110), and Advanced Energy Engineering (D31030070)